Systems and Synthetic Biology Group
The Systems and Synthetic Biology group designs proteins, microbes, and microbial communities for clean energy, manufacturing, agriculture, bioremediation, and human health. We use systems biology approaches to gain a predictive understanding of complex microbes/microbial communities and uncover foundational design rules that govern system level behavior. We employ synthetic biology approaches to redesign proteins and microbial pathways that sequester target critical metals, resist mutational inactivation, and generate products of strategic importance. Using engineering and material science principles, we translate fundamental science discoveries into platform technologies that benefit national security.
Research Area Highlights
-
Prolonging genetic circuit stability through adaptive evolution of overlapping genes
-
Enhanced rare-earth separation with a metal-sensitive lanmodulin dimer
-
Comparison of kill switch toxins in plant-beneficial Pseudomonas fluorescens reveals drivers of lethality, stability, and escape
-
Subsurface microbial communities as a tool for characterizing regional-scale groundwater flow
-
Characterization of exometabolites from a diverse suite of microalgae
-
A Protein-Based Process for Recovery and Separation of Rare Earth Elements
-
Bacterial response to spatial gradients of algal-derived nutrients in a porous microplate
-
LLNL Biosecurity SFA: Secure and Robust Biosystems Design at Sequence, Cell and Population Levels
-
Combinatorial sensor design for selective environmental detection of uranium
-
Microbe encapsulation for selective rare-earth recovery from electronic waste leachates
-
Using engineered bacteria to recover rare earth elements
-
Multicomponent signaling pathway governing an extracytoplasmic stress response in Caulobacter
-
Biogeochemistry of actinides
-
Encapsulin carrier proteins for enhanced expression of antimicrobial peptides
-
Attachment between heterotrophic bacteria and microalgae influences symbiotic microscale interactions
-
Light influences utilization of extracellular organic C and N in cyanobacteria
Prolonging genetic circuit stability through adaptive evolution of overlapping genes
The development of synthetic biological circuits that maintain functionality over application relevant timescales remains a significant challenge. Here, we employed synthetic overlapping sequences in which one gene is encoded or “entangled” entirely within an alternative reading frame of another gene. In this design, the toxin-encoding relE was entangled within ilvA, which encodes threonine deaminase, an enzyme essential for isoleucine biosynthesis. We found that the selection pressure to maintain functional IlvA stabilized the production of burdensome RelE for over 130 generations, which compares favorably with the most stable kill-switch circuits developed to date. This stabilizing effect was achieved through a complete alteration of the allowable landscape of mutations such that mutations inactivating the entangled genes were disfavored. Instead, the majority of lineages accumulated mutations within the regulatory region of ilvA. By reducing baseline relE expression, these more ‘benign’ mutations lowered circuit burden, which suppressed the accumulation of relE inactivating mutations, thereby prolonging kill-switch function. Overall, this work demonstrates the utility of sequence entanglement paired with an adaptive laboratory evolution campaign to increase the evolutionary stability of burdensome synthetic circuits.
Enhanced rare-earth separation with a metal-sensitive lanmodulin dimer
Technologically critical rare-earth elements are notoriously difficult to separate, owing to their subtle differences in ionic radius and coordination number. The natural lanthanide-binding protein lanmodulin (LanM) is a sustainable alternative to conventional solvent-extraction-based separation. In collaboration with Joseph Cotruvo and Amie Boal at Penn State, we identified and characterized a new LanM, from Hansschlegelia quercus (Hans-LanM) with an oligomeric state sensitive to rare-earth ionic radius. X-ray crystal structures illustrate how picometre-scale differences in radius between lanthanum(III) and dysprosium(III) are propagated to Hans-LanM’s quaternary structure through a carboxylate shift that rearranges a second-sphere hydrogen-bonding network. Comparison to the prototypal LanM from Methylorubrum extorquens reveals distinct metal coordination strategies, rationalizing Hans-LanM’s greater selectivity within the rare-earth elements. Structure-guided mutagenesis of a key residue at the Hans-LanM dimer interface modulates dimerization in solution and enables single-stage, column-based separation of a neodymium(III)/dysprosium(III) mixture to >98% individual element purities. This work showcases the natural diversity of selective lanthanide recognition motifs, and it reveals rare-earth-sensitive dimerization as a biological principle by which to tune the performance of biomolecule-based separation processes.
Kill switches provide a biocontainment strategy in which unwanted growth of an engineered microorganism is prevented by expression of a toxin gene. Because kill switches often exploit genetic parts orthogonal to host cell machinery, these circuits have an immense genetic design space for environmental applications. However, kill switches rapidly lose killing function over time because they are not essential and, despite careful design, incur a high fitness cost on the host cell. To inform development of stable yet robust kill switches in relevant chassis strains, we comparatively assess the cell-killing activity of several different toxin systems from the same genetic context in the agriculturally relevant strain Pseudomonas fluorescens. This study unveils a critical tradeoff between lethality and long-term genetic stability. We ultimately propose chassis-specific genetic engineering methods for balancing killing or evolutionary stability to achieve sufficient application-specific containment.
Subsurface microbial communities as a tool for characterizing regional-scale groundwater flow
Remediation of subsurface sites requires an understanding of the underlying hydrogeochemical processes to assess the risks associated with contaminants. Several approaches and models are used to characterize subsurface sites, with particular focus on potential transport of contaminants via groundwater contaminant plumes. As such, the toolkit for monitoring the hydrogeochemical processes of groundwater systems includes water-level and water-quality monitoring. However, groundwater systems also are impacted by subsurface microbial communities, which may help inform groundwater-flow paths, connectivity, and water quality. In this study, we examined the microbial communities of the Death Valley Regional Flow System (DVRFS), a 100,000 km2 region that includes the Nevada National Security Site (NNSS, formerly Nevada Test Site). Samples were collected from 36 locations in three DVRFS groundwater basins: Pahute Mesa–Oasis Valley (PMOV), Ash Meadows (AM), and Alkali Flat–Furnace Creek Ranch (AFFCR). Overall, the DVRFS groundwater microbiome supported the following documented flow-paths with hydraulic connections: (1) Spring Mountains to Ash Meadows; (2) Frenchman Flat and Yucca Flat to Amargosa Desert; and (3) Amargosa Desert to Death Valley. This study demonstrates the utility of combining microbial data with hydrogeochemical information to comprehensively characterize groundwater systems.
Characterization of exometabolites from a diverse suite of microalgae
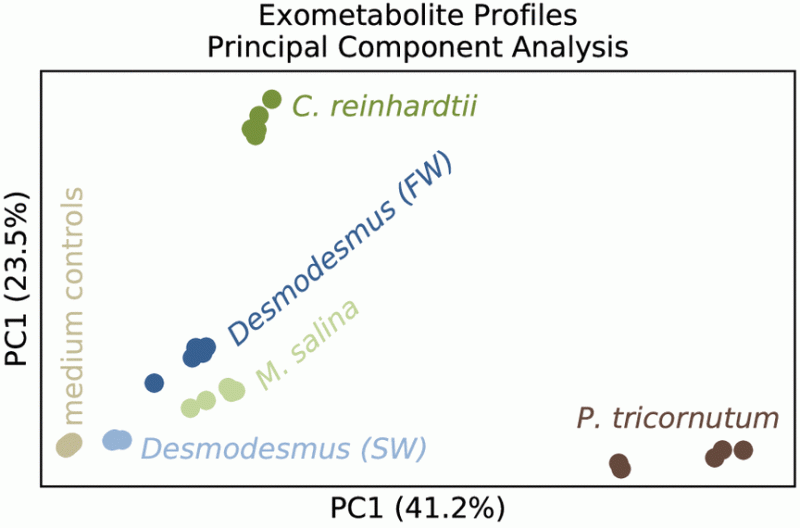
Algal exometabolites are important mediators of algal-algal and algal-bacterial interactions that ultimately affect algal growth and physiology. In a recently published manuscript, µBiospheres SFA scientist Vanessa Brisson led a study which sought to identify effector metabolites involved in interactions within or between algal strains. She profiled exometabolomes across marine and freshwater algae and observed that while phylogeny played a role in exometabolome content, environmental conditions or habitat origin (freshwater versus marine) were also important. She also found that several of these compounds can influence algal growth (as measured by chlorophyll production) when provided exogenously, highlighting the importance of characterization of these novel compounds and their role in microalgal ecophysiology.
Bridging Hydrometallurgy and Biochemistry: A Protein-Based Process for Recovery and Separation of Rare Earth Elements
The extraction and subsequent separation of individual rare earth elements (REEs) from REE-bearing feedstocks represents a challenging yet essential task for the growth and sustainability of renewable energy technologies. As a step toward overcoming the technical and environmental limitations of current REE processing methods, we demonstrate a biobased, all-aqueous REE extraction and separation scheme using the REE-selective lanmodulin protein. Lanmodulin was conjugated onto porous support materials to enable tandem REE purification and separation under flow-through conditions. Immobilized lanmodulin achieved high-purity separation of the clean-energy-critical REE pair Nd/Dy and transformed a low-grade leachate (0.043 mol % REEs) into separate heavy and light REE fractions (88 mol % purity of total REEs) in a single column run. This ability to achieve, for the first time, tandem extraction and grouped separation of REEs from very complex aqueous feedstock solutions without requiring organic solvents establishes this lanmodulin-based approach as an important advance for sustainable hydrometallurgy.
From Sequence to Cell to Population: Secure and Robust Biosystems Design for Environmental Microorganisms
The LLNL Biosecurity SFA seeks to design multilayered containment strategies to safeguard the deployment of engineered microbes in the rhizosphere. Genetically engineered microorganisms (GEMs) play an important role in building and maintaining a sustainable bioeconomy. Without sufficiently robust biocontainment strategies, however, technology adoption and public trust will remain low. To reduce the risk of unintended ecological consequences from environmentally deployed GEMs, the Secure Biosystems Design SFA at LLNL is developing built-in security mechanisms that ensure GEMs function where and when needed. Efforts are concentrated on establishing robust, generalizable biocontainment strategies in environmentally relevant soil microbes at the DNA sequence, cellular, and population levels to achieve containment without sacrificing microbial fitness. Leveraging LLNL’s high performance computing (HPC) and high-throughput gene-editing capabilities, a specific focus of this SFA is on advancing a synthetic gene entanglement strategy for containment. In this strategy, two genes are encoded as overlapping sequences within the same DNA molecule, taking advantage of the fact that the same DNA sequence can be decoded in different reading frames. This DNA sequence overlap protects engineered functions against mutational inactivation and mitigates the potential transfer of engineered genes to naturally occurring microbes. Building on this layer of genetic stability, additional containment strategies that control cellular physiology and direct population coordination are incorporated to increase the overall system robustness to environmental fluctuations. Leveraging LLNL’s rich experience in soil microbial ecology, this SFA evaluates the ecological benefits of these containment mechanisms in soil and rhizosphere environments. By increasing the genomic stability of GEMs and preventing the transfer of potentially invasive traits to native microbiomes, the ultimate objective is to achieve robust and secure niche-specific function of GEMs for safer and more effective environmental applications.
Combinatorial sensor design for selective environmental uranium detetection
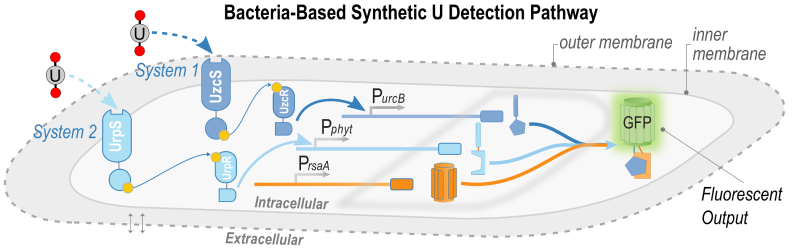
The ability to detect uranium (U) through environmental monitoring is of critical importance for informing water resource protection and nonproliferation efforts. Whole-cell biosensors—microbial cells that are engineered to output a quantifiable signature in response to a stimulus of interest—offer potential for low-cost, in situ analyte detection given their low equipment/energy requirements, ease of operation, minimal sample processing, and potential to function autonomously. We are developing whole-cell sensors for the detection of U and other byproducts of the nuclear fuel cycle. Using a systems biology approach, we identified two previously uncharacterized regulatory pathways—UzcRS and UrtAP—that are responsible for mediating U-dependent gene regulation in C. crescentus. Both pathways exhibit distinct, but imperfect selectivity profiles and function independently with respect to U perception. Using a combinatorial approach, we developed a genetic circuit that triggers GFP expression when the cell receives input from both pathways. The resulting whole-cell sensor enables the selective detection of U in ground water samples.
Microbe encapsulation for selective rare-earth recovery from electronic waste leachates
A. Brewer, A. Dohnalkova, V. Shutthanandan, L. Kovarik, E. Chang, A. M. Sawvel, H. E. Mason, D. Reed, C. Ye, W. F. Hynes, L. N. Lammers, D. M. Park, and Y. Jiao. (2019) Environmental Science and Technology.
A flow-through, biosorption-based rare earth element (REE) extraction technology was developed by encapsulating a bacterium engineered for enhanced REE adsorption within poly(ethylene glycol) diacrylate hydrogels and using the resulting microbe beads as the stationary phase resin in a packed bed column. The microbe bead columns exhibited high stability over multiple REE extraction cycles and enabled the selective extraction of REEs from an electronic waste leachate (NdBFe magnets from cell phone speakers) in a single column extraction step.
Bioadsorption of rare earth elements through cell surface display of lanthanide binding tags
D. M. Park, D. W. Reed, M. C. Yung, A. Eslamimanesh, M. M. Lencka, A. Anderko, Y. Fujita, R. E. Riman, A. Navrotsky, Y. Jiao (2016) Environmental Science and Technology
Rare earth elements (REE) are widely and increasingly used in renewable-energy, consumer, and defense applications, but supplies are vulnerable. We are developing an innovative biotechnology to sequester and recover rare earth elements from low-grade sources (e.g., mine tailings, geothermal fluids, and recyclable materials). We are advancing a cost-effective approach that relies on environmentally-safe microorganisms (patent pending). We combine native cell physiology with advanced synthetic biology to recover rare earth elements through biosorption. Our engineered microbes exhibit enhanced adsorption capacity and selectivity towards rare earth metals even in the presence of high concentrations of competing metals.
The UzcRS two‐component system in Caulobacter crescentus integrates regulatory input from diverse auxiliary regulators
D. M. Park, K. W. Overton, and Y. Jiao (2018) Molecular Microbiology
Multicomponent signaling pathway governing an extracytoplasmic stress response in Caulobacter. TheUzcRSTCS is the core signaling unit of this multicomponent signaling pathway and functions as a U/Zn/Cu‐dependent global activator of genes encoding extracytoplasmic functions. Arrows are colored based on interactions that occur under normal conditions of growth conditions (i.e. no added inducer; black) or following exposure to U/Zn/Cu (blue) or an unidentified stressor/s (magenta). Dashed lines represent either indirect interactions or unconfirmed direct interactions, and dashed arrows for UzcR reflect a uzcR‐dependent change in transcription, but no upstream ChIP‐seq peak.
Biogeochemistry of Actinides
Contacts: Nancy Merino and Yongqin Jiao
Microorganisms influence actinide environmental fate and transport through several mechanisms, such as bioaccumulation, biosorption, and biomineralization. However, the effect of microbial products on actinide mobility remains largely unexplored. At Savannah River Site (SRS) Pond B in South Carolina, we are investigating the role of microbial intracellular and extracellular products on actinide partitioning (Pu, Am, Cs) between the water column and sediments. Pond B received nuclear reactor cooling water between 1961–1964. We are currently measuring several biogeochemical parameters of Pond B over a 1-year period and conducting microcosm experiments under different conditions to ascertain whether specific metabolisms result in different microbial exudates that affect Pu partitioning between the sediment-water interface.
Encapsulin carrier proteins for enhanced expression of antimicrobial peptides
T-H. Lee, T. S. Carpenter, P. D’haeseleer, D. F. Savage, and M. C. Yung. (2019) Biotechnology and Bioengineering, Editor’s Choice Article
Antimicrobial peptides (AMPs) are regarded as attractive alternatives to conventional antibiotics, but their production in microbes remains challenging due to their inherent bactericidal nature. We have engineered a cage‐disrupted encapsulin carrier protein containing multiple TEV protease recognition sites. When fused to AMPs, this engineered encapsulin protein enabled robust AMP expression in Escherichia coli. In contrast to cage‐forming encapsulin proteins, release of AMP from the cage‐disrupted protein by TEV protease was highly efficient, ultimately yielding highly active AMP.
See also: M. C. Yung, F. A. Bourguet, T. S. Carpenter, M. A. Coleman (2017) Microbial Cell Factories
Attachment between heterotrophic bacteria and microalgae influences symbiotic microscale interactions.
T. J.Samo, J. A. Kimbrel, D. J. Nilson, J. Pett-Ridge, P. K. Weber, X. Mayali (2018) Environmental Microbiology
NanoSIP images and data of the alga Phaeodactylum tricornutum, Haliscomenobacter relative, and bacteria showing atom percent excess in phycosphere enrichment. The elongated and 13C‐enriched cells of P. tricornutum (red, orange, yellow) in the left image can be seen among the less enriched bacteria (blue) while filamentous cells of 15N‐enriched Haliscomenobacter relative in the middle image can be seen among the smaller bacteria and alongside the algae in the merged RGB image on the right.
Light regimes shape utilization of extracellular organic C and N in a cyanobacterial biofilm
R. K. Stuart, X. Mayali, A. Boaro, A. Zemla, R. C. Everroad, D. Nilson, P.K. Weber, M. Lipton, B. Bebout, J. Pett-Ridge, M. P. Thelen (2016) mBio
Representative NanoSIMS images of cyanobacterium ESFC-1 trichomes and heterotrophs 24 h after addition of 13C-labeled and 15N-labeled extracellular organic matter. Trichomes and associated microbes were identified morphologically by scanning electron microscopy prior to NanoSIMS analysis. Left, images (panels A and C and panel E) are paired images from a light-treated biofilm; Right, images (panels B and D and panel F) are paired images from a dark-treated biofilm. (A and B) Scanning electron microscope images taken prior to NanoSIMSanalysis. White outlines in panels C to F correspond to areas analyzed (ROIs), from which values for individual cells are derived. (C and D) 13C enrichment images. (E and F) 15N enrichment images. APE, atom percent excess.